Nuclear Fusion: The Quest to Harness Stellar Power on Earth
Written on
Chapter 1: A Journey to the Sun
Let's embark on an imaginative expedition to our sun.
To grasp the scale of this journey, visualize Earth reduced to the size of a pebble. In this scenario, the sun would appear as a basketball, illustrating the immense difference in size.
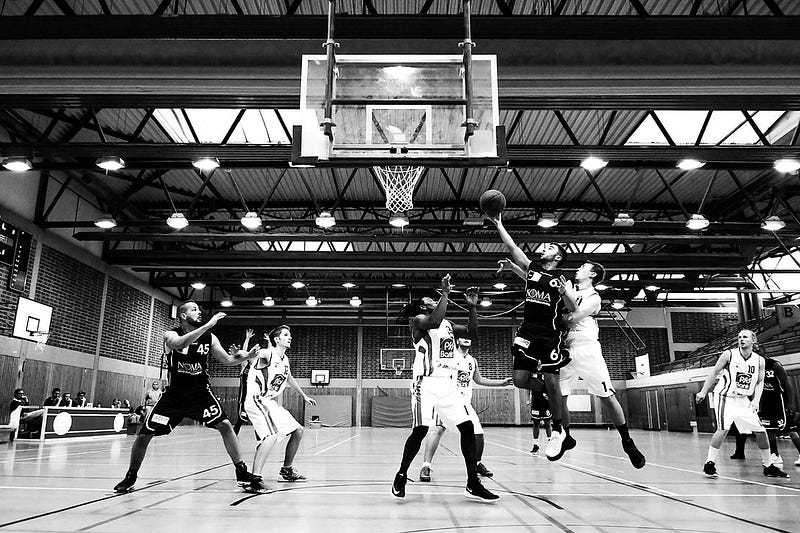
If we were to travel from our pebble-sized Earth positioned at one end of a basketball court, the basketball-sized sun would be located at the opposite end, just beneath the hoop.
Upon examining the sun more closely, we see a luminous sphere of gas. The outer layer we observe is known as the Photosphere. If we could cut the sun open, akin to slicing an onion, we would discover it has four distinct layers. Our primary focus is the core at the sun's center, where extreme heat and immense pressure from its size force atomic nuclei to merge (or fuse), releasing energy in the process.
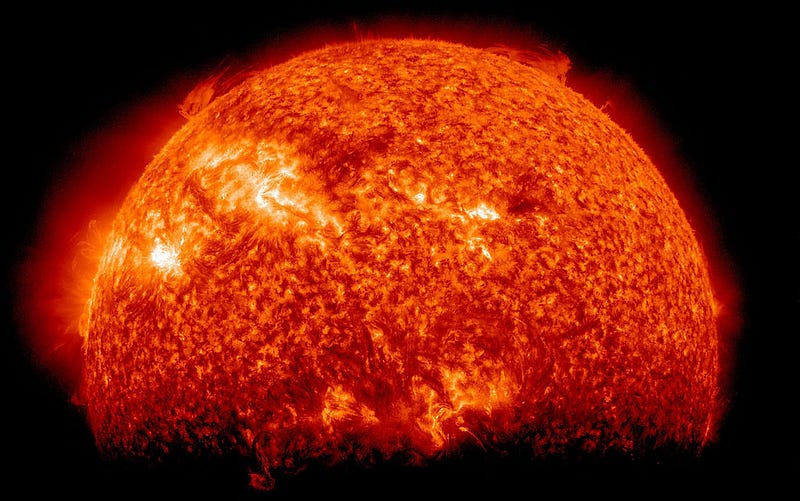
The process occurring in the sun’s core is nuclear fusion, which is responsible for the sun's seemingly limitless energy supply. But can we replicate this phenomenon on Earth?
What is Nuclear Fusion?
Nuclear fusion involves the merging of two lighter atoms under extreme temperatures to form a heavier atom, accompanied by a significant release of energy. The sun and stars exemplify this process.
Before we delve deeper, let’s visualize the scale of an atom and its nucleus. Imagine a single grain of sand in your hand, then expand it to the size of Earth—this is a challenging image to conjure. Next, zoom in on this Earth-sized grain until you find an average two-story house, representing the size of a single atom within it.
For a more tangible understanding, picture a football stadium—like Wembley in London. If this stadium symbolizes a hydrogen atom, a grain of sand at its center would represent the hydrogen nucleus, a mere speck in a space designed for 90,000 spectators.
Returning to our original scale, a single grain of sand contains approximately 43 billion billion billion atoms. This illustrates the minuscule scale we must work with to achieve nuclear fusion.
I won’t delve into excessive technical detail here; several informative videos can clarify the concepts further. However, it’s essential to understand that the nucleus of an atom carries a positive charge. When similarly charged nuclei approach one another, they repel due to the Coulomb Barrier—much like opposing magnets.
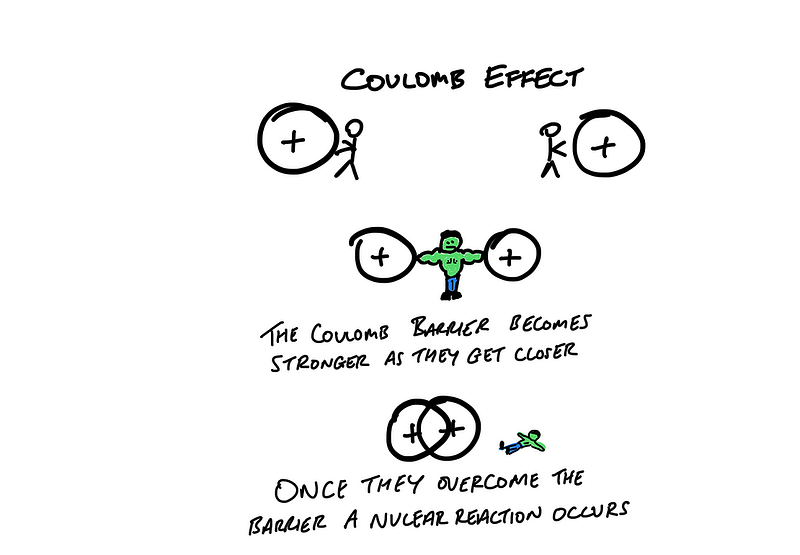
To overcome this barrier, we need sufficient heat and/or pressure to facilitate the fusion of these nuclei, releasing substantial energy. The sun achieves this through its intense heat and gravitational force, while we can replicate it on Earth by generating extreme temperatures or colliding nuclei together.
While the idea of creating mini-suns on Earth to power our lives sounds promising, the reality is that this process currently consumes more energy than it produces.
The Holy Grail of Energy
The quest to "bottle the sun" has been ongoing for nearly 85 years. The first attempts were made at the NACA Langley Research Center in 1938, which did not succeed in achieving nuclear fusion.
Progress was minimal until 1958, when the ZETA device in Harwell, UK, initially claimed success but later retracted the statement. That same year, Scylla I at Los Alamos, New Mexico, achieved the first controlled thermonuclear fusion in a lab. Although this was a milestone, the method used was quickly deemed impractical for scaling up to a working reactor.
Below is a timeline detailing the advancements in nuclear fusion by decade for further reference. As of 2022, we still have yet to achieve commercial-scale nuclear fusion, and experts predict we remain at least ten years away.
We have successfully created plasma multiple times, but achieving a net energy gain (Q) has eluded us.
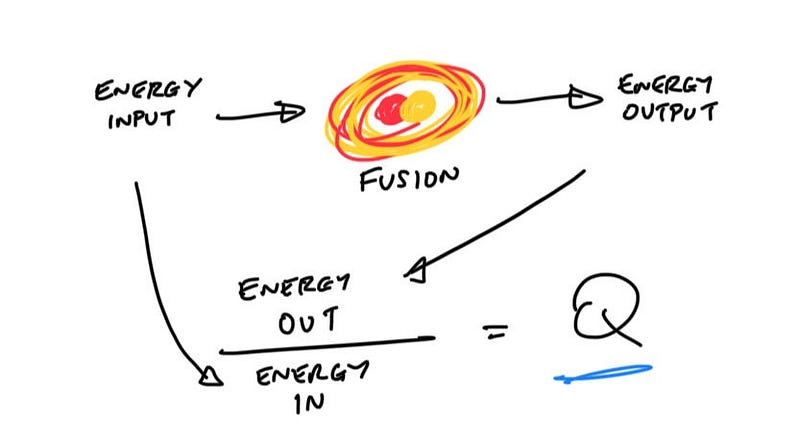
The highest energy gain recorded for a long time was 0.67 by JET in the UK, recently surpassed by the US Department of Energy’s National Ignition Facility in California with a Q of 0.7, albeit for only brief intervals. JET also reported a Q of 0.33 sustained for 5 seconds.
It's important to note that the Q calculation is often debated. The figures are based on the energy required to generate plasma, but using the total energy needed to operate the facility would yield significantly lower Q values.
Nonetheless, we are still far from achieving nuclear fusion at a sustainable level suitable for commercial use.
Summary: The Future of Nuclear Fusion
Nuclear fusion is the process of merging atomic nuclei to create a single, heavier nucleus, generating vast amounts of energy. Scientists have been striving to harness nuclear fusion as a clean and efficient energy source for many years.
Fusion can be maintained by generating extremely high temperatures and pressures, and the energy produced can be transformed into electricity. This method has the potential to be much cleaner and more efficient than current nuclear power technologies. However, substantial challenges remain before fusion can be commercialized, such as developing materials that can endure the necessary extreme conditions and managing radioactive waste.
Key advantages of nuclear fusion include its cleanliness, safety, and lack of greenhouse gas emissions. The potential for large-scale energy production makes it an attractive alternative to fossil fuels. Yet, the hurdles associated with nuclear fusion suggest it may be years before we see widespread commercial systems. Research continues to progress towards this goal, providing optimism for a more sustainable future.
While the path ahead for nuclear fusion remains uncertain, it holds promise as a vital component of the future energy landscape.
Nuclear Fission vs Fusion
Both nuclear fusion and fission are processes that generate energy from atoms. As mentioned earlier, fusion occurs when lighter atoms combine, whereas fission involves splitting a heavier atom. Both methods yield substantial energy.
The primary differences between nuclear fusion and fission lie in their fuel types, by-products, energy output, and technological maturity.
- Fuel Types: Fusion uses hydrogen, while fission relies on uranium or plutonium.
- By-Products: Fusion generates helium, while fission produces spent nuclear fuel, classified as nuclear waste requiring long-term storage.
- Energy Output: Fission generates energy one million times greater than conventional sources like coal, while fusion can release three to four times that of fission.
- Technological Maturity: Fission technology has been in use since the 1950s, with ongoing efforts to enhance safety and efficiency. In contrast, fusion remains the "holy grail" of the nuclear field, with research ongoing since the 1930s, aiming for a functional fusion reactor by 2040.
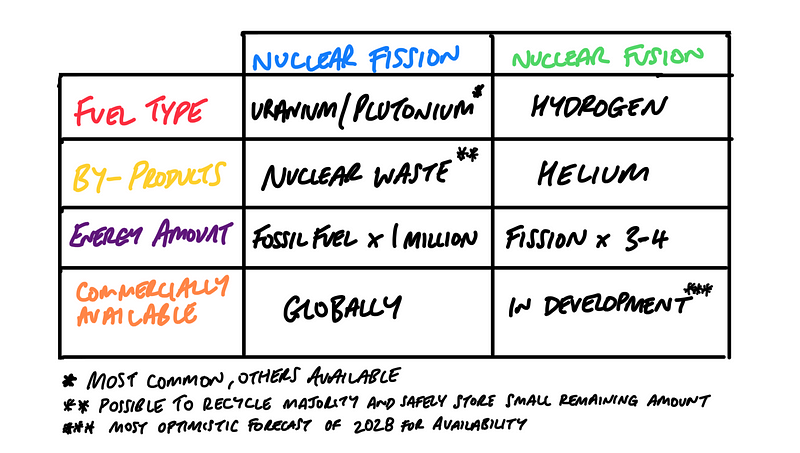
For those interested in more articles like this, consider subscribing to Medium for the price of a coffee each month.
Chapter 2: Exploring Nuclear Fusion Through Video
This video illustrates the process of building a nuclear star in a jar, showcasing the principles of fusion and its potential applications.
This video covers the quest for commercial fusion energy, detailing the advancements and challenges faced in developing this technology.